Background
Inhaltsverzeichnis
Biomass degradation
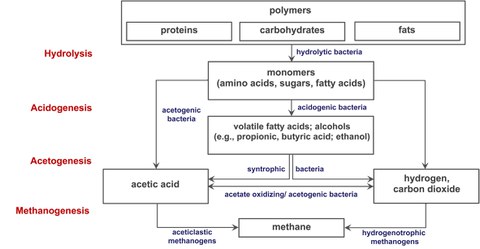
Figure 1: The four steps of methanogenic biomass dedradation
In nature, organic material is degraded, e.g., in anoxic sediments and gastrointestinal tracts of animals, through the process of anaerobic digestion (AD). AD occurs in the absence of exogenous electron acceptors like oxygen, nitrate, or sulfate and a complex microbial community performs this four-step process (Figure 1). After enzymatic hydrolysis of biomass, monomers are fermented to molecular hydrogen (H2), CO2, volatile short-chain fatty acids, and alcohols (primary fermentation); the latter two are substrates for secondary (syntrophic) fermenting microbes, which oxidize fermentation products generating mostly acetate, CO2, and H2; however, this process is only sufficiently exergonic for the organisms to thrive by if the H2 partial pressure is kept very low by syntrophic, H2-consuming microorganisms. Formation of methane, methanogenesis, is the final step of AD and exclusively carried out by methanogenic members of the domain Archaea. The main methanogenic substrates during AD are acetate and H2+CO2, which are converted to methane by distinct but overlapping methanogenic pathways operative in phylogenetically diverse methanogens. H2+CO2 is converted to methane by hydrogenotrophic methanogens, which often serve as the syntrophic “partners” of the syntrophic fermenting microbes. Acetate is methanized by aceticlastic methanogens of which only two genera, Methanosarcina and Methanotrix, are known. Unlike Methanotrix, most Methanosarcina species are also able to grow via hydrogenotrophic methanogenesis but require higher threshold concentrations for H2 than obligate hydrogenotrophic methanogens. Thus, knowledge about the methanogenic community in biogas reactors is of interest in order to understand the biogas process, perturbations of it and, ultimately, maximize methane yields.
Methane
Methane is the most abundant hydrocarbon present in our atmosphere. Its current concentration of >1,900 ppb has tripled since pre-industrial times and an estimated 5 x 1014 g of biologically produced methane annually released into our atmosphere. Methane represents either a highly attractive renewable energy source because aerobic combustion of methane produces only H2O and CO2, or a frightening contribution to global warming because the global warming potential of methane is more than 20 times higher than that of CO2. The only organisms producing (significant amounts of) methane are methanogenic archaea (methanogens). The biology of methanogens is, therefore, a relevant "target" to tackle climate change (see ASM report and news coverage). In anaerobic environments lacking abundant electron acceptors such as sulfate or nitrate methane is the final breakdown product of organic matter. Thus, methanogenesis, the biological formation of methane, plays an essential role in the global carbon cycle because it recycles organic matter from anaerobic to aerobic environments. Importantly, methanogens comprise one of the few experimentally tractable groups among the Archaea and, thus, provide outstanding model organisms for the study of this so-called "third form of life". Archaea were recognized as a distinct phylogenetic group only 30 years ago and were initially renowned for being strictly anaerobic and/or inhabiting inhospitable environments like solfataric hot springs, soda lakes, and submarine volcanic vents. However, it is now apparent that Archaea are ubiquitous and constitute a significant portion of the global biomass.
Methanogenesis
All methanogenic archaea investigated to date strictly rely on methanogenesis for energy conservation and, thus, growth. Principally, methanogenic substrates are converted to methane with the concomitant generation of an ion motive force across the cytoplasmic membrane, which can then be employed for ATP synthesis or other energy requiring cellular processes. The number of substrates utilized for methanogenesis is quite limited reflecting the narrow ecological niche methanogens occupy: most methanogens are only able to grow with H2 + CO2 (or formate; via the hydrogenotrophic path), some can utilize methylated compounds like methanol and methylamines (via the methylotrophic path), and some can grow with acetate (via the aceticlastic path). These different substrate classes are metabolized via three distinct, but overlapping, pathways of methanogenesis (Figure 2).
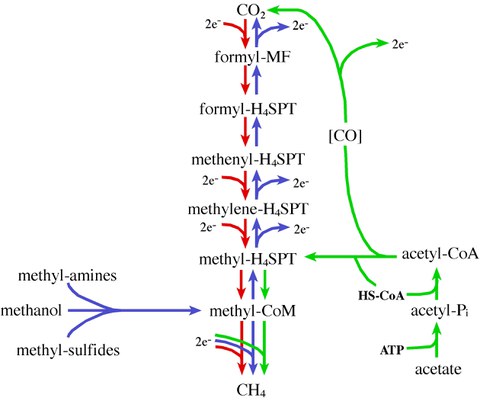
Figure 2: The different paths of methanogenesis. The hydrogenotrophic (red), the methylotrophic (blue), and the aceticlastic (green) pathway.
Hydrogenotrophic methanogenesis, which is carried out by most methanogens, involves the stepwise reduction of CO2 to methane via coenzyme-bound intermediates with reducing equivalents derived from the oxidation of H2 (Figure 2, red arrows). In the methylotrophic pathway (Figure 2, blue arrows), methylated compounds such as methanol, methylamines and methylsulfides are funneled into the methanogenic pathway by methyl-transfer to coenzyme M (CoM). Methyl-CoM is subsequently disproportionated in a 3:1 ratio; one mole of methyl-CoM is oxidized to CO2 for every three moles of methyl-CoM reduced to methane. Methyl-CoM oxidation occurs through a reverse of the CO2 reduction pathway, generating reducing equivalents for methyl reduction. Aceticlastic methanogenesis (Figure 2, green arrows) proceeds by the activation of acetate to acetyl-CoA. Subsequently, the bifunctional carbon monoxide dehydrogenase/acetyl-CoA synthase (CODH/ACS) complex splits acetyl-CoA into enzyme-bound CO, a methyl-group, and free coenzyme A. CODH/ACS donates the methyl-group to tetrahydrosarcinapterin (H4SPT) and methyl-H4SPT is reduced via reactions of the hydrogenotrophic pathway (see above). The CO produced in the CODH/ACS-catalyzed reaction is oxidized to CO2 generating the electrons needed for reduction of the methyl-group to methane. All methanogens known use cofactor F420 (a 5-deazaflavine derivative functionally analogoous to NAD+), which results in a bright blue-green autofluorescence of the cells when illuminated at 420 nm (Figures 3 and 4).
Methanogens
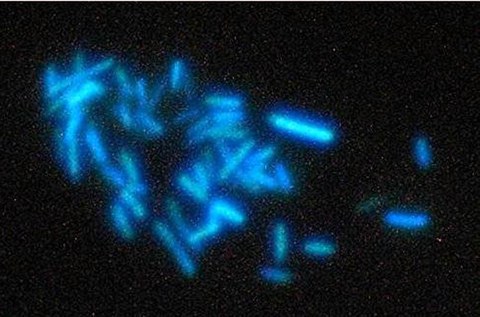
Figure 3: A hydrogenotrophic methanogen
As most methanogens (e.g., Methano-bacteriales and Methanococcales) are only able to grow with H2 + CO2 (Figure 2, red arrows), they are obligate hydrogenotrophs. Obligate hydrogenotrophs lack cytochromes and a "classical" respiratory chain but employ, beside generation of a sodium motive force at the step of methyl-transfer from tetrahydromethanopterin (similar to H4SPT) to CoM, flavin-based electron bifurcation for energetic coupling.
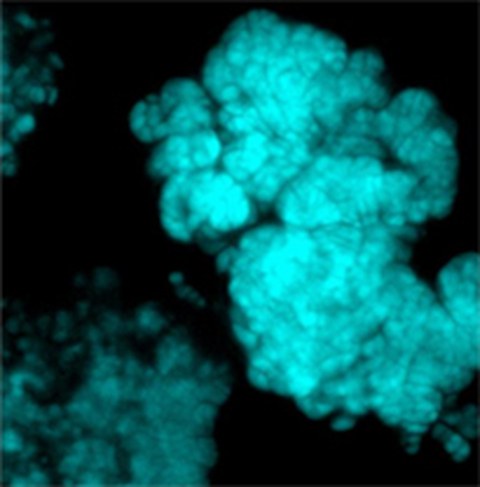
Figure 4: Methanosarcina flavescens
Methylotrophic and aceticlastic members of the Methanosarcinales employ cytochrome-containing respiratory chains and methanophenazine, a membranous electron carrier functionally anaolous to quinones. Evolutionary, these methogens may be considered as "modern" compared to the more "archaic" hydrogenotrophs.