Current research
Table of contents
- Genetic analysis in methanogenic archaea
- Methanosarcina - a model species for genetic analysis of methanogenesis
- Adapting genetic methods for use in methanogens
- Analysis of the carbon monoxide metabolism in Methanosarcina acetivorans
- Mechanism and regulation of selenoprotein synthesis in Archaea
- Expanding the substrate range of methanogenic archaea
- Methanogenic hydrogen metabolism in biogas plants (completed)
- Producing isoprenoids in Methanosarcina acetivorans
Genetic analysis in methanogenic archaea
Members of the domain Archaea mediate all significant biological methane production on earth. Studies on methanogenesis have produced a wealth of novel biochemical knowledge due to the unique nature of methanogenic metabolism and although the basic biochemistry of methane production has been elucidated, relatively little is known about other aspects of the methanoarchaea, many of which have direct relevance to the process of methanogenesis. This lack of understanding encompasses nearly all aspects of the growth, metabolism and physiology of these unique and important prokaryotes. One reason for this lack of understanding is the extreme sensitivity of methanogens towards oxygen necessitating elaborate methods and equipment for their maintenance. Another significant factor that has contributed to this lack of knowledge is the dearth of genetic methods that can be applied to the problem. However, this has changed recently and new methods now make genetic analysis of methanogenic archaea possible.
Methanosarcina - a model species for genetic analysis of methanogenesis
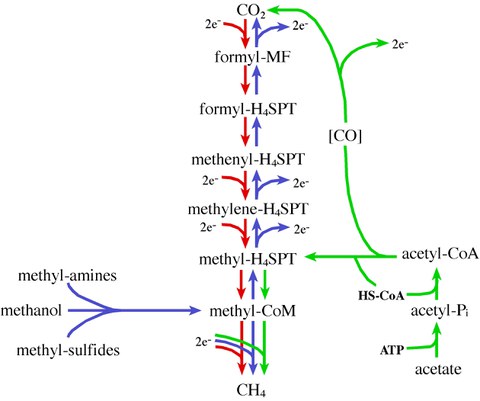
Figure 1: The different paths of methanogenesis. The hydrogenotrophic (red), the methylotrophic (blue), and the aceticlastic (green) pathway.
While most methanogens have only a single methanogenic pathway and can thus grow on only one or two substrates, Methanosarcina species are more metabolically versatile and can use H2/CO2, methanol, methylamines, methylsulfides, and acetate as substrates for methanogenesis (Figure 1). This capacity to utilize alternative routes for methanogenesis makes Methanosarcina particularly attractive for genetic analysis of methanogenesis itself because disruption of one of the pathways still allows for isolation of viable mutants capable of growth by means of one of the other pathways. In this regard, Methanosarcina species are the only methanogenic organisms for which the dual requirements of metabolic diversity and proven genetic techniques are satisfied. Also, the availability of complete or nearly complete genome sequences from three Methanosarcina species facilitates holistic approaches like proteomic and transcriptomic analyses.
Adapting genetic methods for use in methanogens
Despite the past progress, genetic analysis of methanogens is generally still not a routine approach. Many powerful techniques, which are commonly applied for other organisms have not yet -or only recently- been adapted for genetic analysis of methanogens.
- As most commonly used reporter systems (e.g., green fluorescent protein, beta- galctosidase) require oxygen, we developed beta-lactamase as a reporter enzyme for Methanosarcina and Methanococcus to allow color-screening of mutant libraries under anaerobic conditions.
- Together with the group of B. Suess (TU Darmstadt, Germany) a tetracycline-responsive riboswitch was developed for Methanosarcina to allow inducible gene expression without additional trans-acting factors.
- A random in vivo mutagenesis designed for Methanosarcina was adapted for use in Methanococcus maripaludis and used to identify genes involved in formate-dependent growth.
Analysis of the carbon monoxide metabolism in Methanosarcina acetivorans
Besides methanol, methylamines, and acetate, Methanosarcina acetivorans can also use carbon monoxide (CO) as the sole energy source. Surprisingly, CO is not converted to methane only but also to acetate, formate, and methyl-sulfides, which is unusual for a methanogen. Acetate is formed via a pathway analogous to that found in acetogenic bacteria (Wood-Ljungdahl pathway) and is presumably coupled to energy conservation. Thus, M. acetivorans can grow both acetotrophically and acetogenically. For CO-dependent dimethyl-sulfide formation, fused corrinoid/methyltransfer proteins were shown to be responsible.
On the other hand, neither the role of the formate nor the mechanism by which it is produced is currently understood. As to CO utilization, four putative carbon monoxide dehydrogenases (two as CODH/ACS) encoded in the M. acetivorans genome are involved in oxidation of CO and/ or generation of acetate. We are studying the CO metabolism of M. acetivorans by means of physiological analysis driven by genetic manipulation. Creating mutants affected in their CO metabolism and determining their physiological, molecular, and biochemical characteristics will not only help to understand the processes by which this toxic gas is utilized, but also expand our knowledge of processes necessary for sensing of, and adaptation to, different growth substrates which is the basis for the metabolic flexibility of Methanosarcina species.
Mechanism and regulation of selenoprotein synthesis in Archaea
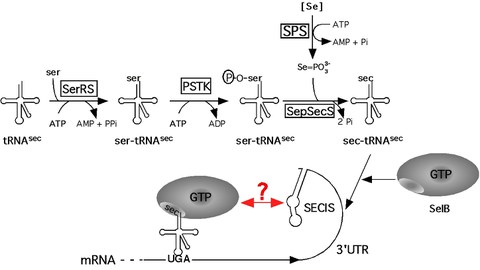
Figure 2: Model of the pathway of selenoprotein synthesis in archaea.
Selenium, which is an essential trace element in many organisms, is found in the catalytic site of numerous redox-active enzymes in the form of selenocysteine within all three domains of life. It was designated the 21st genetically encoded amino acid because it is co-translationally inserted into growing polypeptides and universally encoded by the opal (stop-) codon UGA on the mRNA. For its insertion, a signal on the mRNA (the selenocysteine insertion sequenceor SECIS element) is required to recode the nonsense- into a sense codon. The pathway of selenocysteine biosynthesis and incorporation has been exhaustively studied in E. coli; however, it is apparent now that selenoprotein synthesis in Bacteria differs significantly from that in Archaea (Figure 2) and Eukarya. For example, the SECIS element is located adjacent to the UGA codon in Bacteria, whereas in Archaea and Eukarya, it is not part of the structural gene but located in the non-translated region of the selenoprotein mRNA. This raises the important question how communication between the site of recoding (the SECIS element) and the site of translation (the ribosome) is established and regulated. Furthermore, the conversion of serine to selenocysteine (both bound to the selenocysteine-specific tRNA) is known to occur in Archaea and Eukarya via a phosphorylated intermediate. However, its physiological role has not been elucidated in either domain.
We are studying archaeal selenoprotein synthesis and selenium-dependent gene regulation in Methanococcus maripaludis. It is one of the few methanogens for which a facile system for genetic manipulation has been established. Besides the selenoproteins, Methanococcus maripaludis also encodes a set of selenium-free isoforms, which are synthesized during selenium starvation or when the path of selenocysteine biosynthesis and incorporation is disrupted which makes isolation of such mutants feasible. Furthermore, its mesophilic and comparably fast growth to high cell densities, as well as its available genome sequence makes this organism particularly attractive for genetic, physiological, and biochemical analysis.
Expanding the substrate range of methanogenic archaea
All methanogenic archaea investigated to date strictly rely on methanogenesis for growth. The number of substrates utilized for methanogenesis is quite limited reflecting the narrow ecological niche methanogens occupy: most methanogens are only able to grow with H2 +CO2, some can utilize methylated compounds, and some can grow with acetate (Figure 1). However, methanogens need to sustain carbohydrate metabolism for anabolic purposes and some were shown to form glycogen as cellular storage material during nutrient deprivation. Inspection of the M. acetivorans genome sequence revealed the presence of most functions required for glucose oxidation, except for those of carbohydrate uptake and activation. We complemented Methanosarcina with the missing functions for glucose degradation, generating a strain capable of converting glucose to methane. This strain is currently being analyzed and will be used in the future to (i) evolve a strain that reliably forms methane from reduced carbohydrates in order to supplement biogas technology, and (ii) address the ecologically relevant question why no carbohydrate-utilizing methanogen was so far found in the environment.
Methanogenic hydrogen metabolism in biogas plants (completed)
Anaerobic biomass degradation to methane proceeds via a multi-step pathway and involves a plethora of hydrolyzing, fermenting, syntrophic, and methanogenic microorganisms. Molecular hydrogen (H2) is a key molecule during this process. It is formed both during primary and secondary (syntrophic) fermentation of reduced carbon compounds and utilized as electron donor forCO2 reduction to acetate by acetogenic bacteria and to methane by hydrogenotrophic methanogens. Although increasing H2 partial pressure is beneficial for these organisms it must be delicately balanced because syntrophic bacteria only tolerate a low H2 threshold concentration. In order to better the understanding of the biogas process we are investigating H2-dependent methane formation by (i) assessing the diversity and abundance of the microorganisms involved (DGGE, qRT-PCR, and FISH), by (ii) isolating and characterizing the relevant (by abundance and process activity) methanogenic organisms, by (iii) correlating their hydrogenase activity to the total hydrogenase activity, and by (iv) correlating H2 consumption/formation and hydrogenase activity in dependence to reactor performance. With the knowledge gained we aim at a more efficient way to monitor process perturbations for timely remedy in order to increase stability, methane production rate and methane yield in biogas digesters. The project, funded by BMBF (03SF0421C), was completed in 2015.
Producing isoprenoids in Methanosarcina acetivorans
Methanogens generate the isoprenoid side chains of their membrane lipids from acetyl-CoA via a modified MVA pathway. Since M. acetivorans produces high amounts of acetyl-CoA from CO, isoprene and, subsequently, isoprenoids of added value will be produced with M. acetivorans. Single genes encoding eukaryal isoprene synthase and other isoprenoid synthases (IPs) will be introduced into M. acetivorans. Gene expression will be induced using an available inducible system. This research is part of the MethanoPEP project.