Thermal and thermoelectric transport properties down to 50 mK
Conduction of heat in a material via heat currents is a phenomenon analogous to charge transport via electrical currents. Thermoelectric phenomena arise from the entanglement of thermal and electrical transport processes due to (1) heat transport by charge carriers and (2) scattering processes between charge carriers and other heat-carrying quasiparticles, e.g. phonons. By studying the thermal conductivity κ, the thermopower S and the Nernst coefficient N at low temperatures and magnetic fields, we gain valuable information on charge and heat carriers and low energy excitations. Combined with knowledge of the electrical resistance, these measurements give the unique opportunity to disentangle different scattering mechanisms.
Our studies focus on materials with unconventional transport phenomena, such as superconductors, quasi-1D conductors, rare-earth systems, Weyl semimetals, delafossites and quantum magnets.
Millikelvin thermal transport
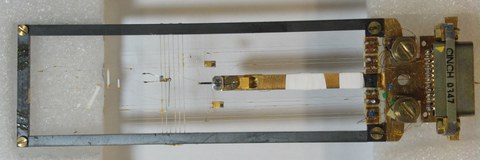
Thermal conductivity setup for dilution refrigerator.
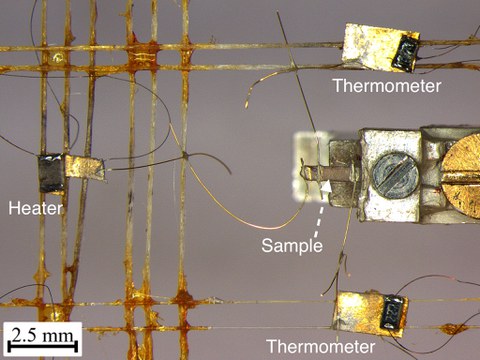
Thermal conductivity setup. The heater is on the left, the two thermometers are on the goldplates at the top and bottom. All of them are thermally connected to the sample via gold wires, but thermally decoupled from the environment via superconducting wires. The sample is thermally anchored to the silver piece screwed onto the coldfinger (center-right).
We are able to perform thermal conductivity, thermopower, and Nernst effect experiments at temperatures ranging from ~30 mK to 5 K, and in magnetic fields, up to 8 T.
Measuring thermal transport properties at temperatures below 1 K requires special experimental techniques. We apply a two-thermometer-one-heater method with RuO chip thermometers and a thin film resistor as heater. This setup is placed in a 3He/4He dilution refrigerator. Mechanical damping of vibrations from the pumps and appropriate electrical shielding and grounding are essential to restrict the heat input into the system.
An important issue for low-temperature thermal transport measurements is the thermal coupling of the sample to the thermometers, the heater, and the bath. This is achieved by evaporating gold pads as contacts directly onto the sample. The sample is clamped mechanically to the bath to further improve the thermal contact.
Another crucial point is the calibration of the sample thermometers, which is not reproducible for consecutive measurements, especially at very low temperatures (<100 mK). Therefore, we perform an in-situ calibration of the sample thermometers against the cold-finger thermometer for each temperature scan.
Thermal transport up to 300 K
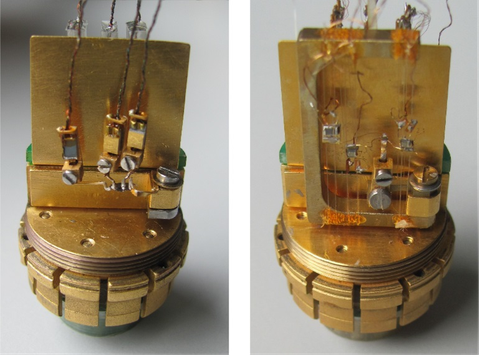
Commercial setup for measuring thermal conductivity and thermopower and a modified setup for smaller samples.
We are using different setups to measure thermal and thermoelectric properties up to 300 K: A commercial PPMS puck, a modified puck for smaller samples as well as a home-built dedicated insert.
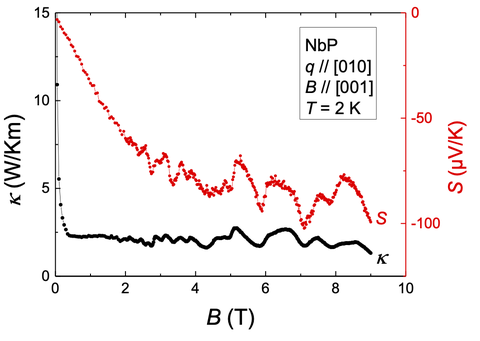
Quantum oscillations in the thermal conductivity and the thermopower of the Weyl semimetal NbP (Stockert 2017).