Neutron Imaging
In comparison to X-ray radiography, neutron imaging resembles a complementary approach as a non-destructive method for screening the components of desired objects. Since the fundamental interaction of neutrons with the material is linked to the nucleus the atom, the trend of the cross sections across the periodic table is not monotonously increasing as for X-ray, which provides different means of identifying elements and in some cases even isotopes. As a consequence, neutrons are able to penetrate heavy and thick materials, were even high-energy and high-intensity X-ray cannot provide any contrast.
A scheme and a CAD-Model of the neutron imaging setup are depicted in Figure 1. The incoming neutrons (n) from the right are indicated by the arrows impinging on a LiF/ZnS:Ag scintillator with a thickness of the coating of 200 µm. The scintillator converts the neutron signal over several steps into a monochromatic photon signal with a wavelength of about 450 nm (blue). The converted signal is then reflected by a coated mirror to the CCD-Detector. That way, the CCD-Detector is not likely to be hit by the radiation field of the reactor. However, noise from the reactor, the environment as well as atmospheric muons and cosmic rays do add to the signal but can be corrected in some post-processing steps. Because of the thickness of the scintillator, an effective resolving power of 266 µm per two pixels with a field of view (FOV) of approximately 136x136 mm has been achieved. The FOV can be arranged up to 155x155 mm.
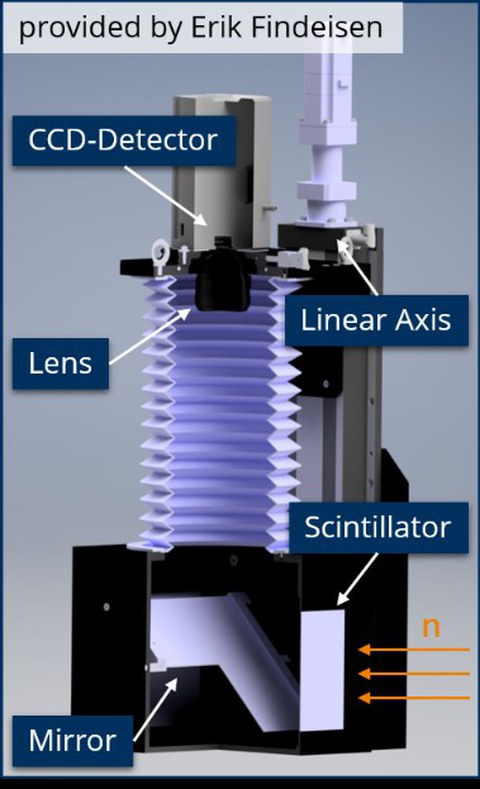
Figure 1: CAD-Model of the neutron imaging setup incl. MidiBox and CCD-Detector
A scheme of the image formation process can be seen in Figure 2. The experimental channel of the reactor provides the neutron beam, which, after collimation, has a specific beam profile. The beam then interacts with the sample to be investigated followed by the scintillator, which is converting the neutron signal. The CCD-Detector collects the light emitted along its 1024x1024 pixels.
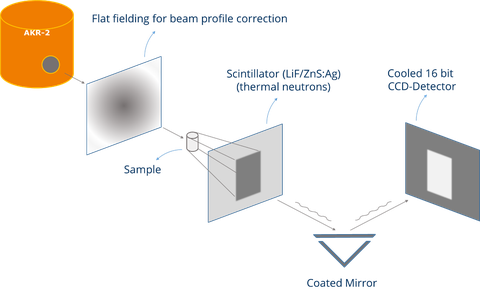
Figure 2: Schematic view of the image formation process
An example application of the neutron imaging is shown in the following. Figure 3 shows the radiographs of a hydrogen battery (HYDROSTICK® PRO) first in a fully loaded and then in a partially emptied state. These images have been made with an old set-up, which was used a proof-of-concept for the feasibility of neutron imaging at low-flux facilities. Lastly, the difference from both states reveals the loaded hydrogen content.
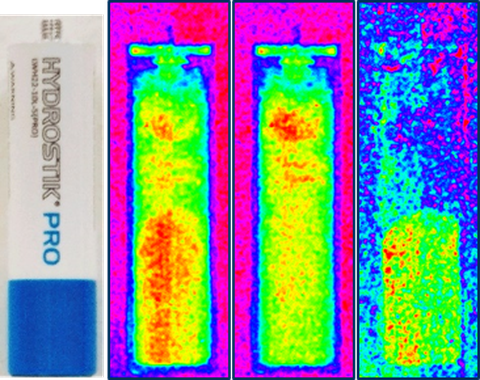
Figure 3: from left to right – image of HYDROSTICK® PRO, fully loaded, almost empty, difference image
For comparison, the results achieved with the new set-up of a similar experiment but with more charge states is shown in Figure 4. The radiographs show the hydrogen storage as well as the mounting bracket or rather the iron screws fixing the aluminum profiles. Using the empty state image as a reference, the changes in hydrogen content can be made visible.
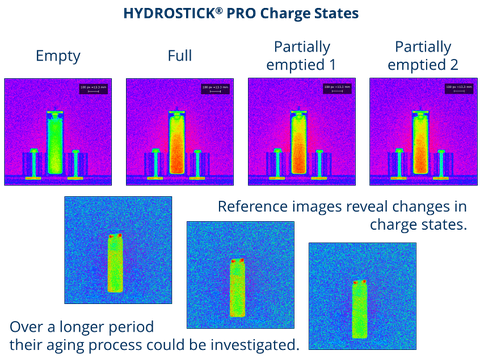
Figure 4: Overview of the radiographies obtained with the new set-up
However, despite the strong noise component when imaging with a low exposure time, even imaging experiments of dynamic processes are possible. In the following a series of the water accumulation of an air-breathing fuel cell is shown. Additionally, the water content in the pictures have been made visible utilizing several strategies like using a denoising filter and setting a threshold, but also more advanced tools have been used like the k-means clustering [1] (unsupervised machine learning) and the Weka tool [2] (supervised machine learning).
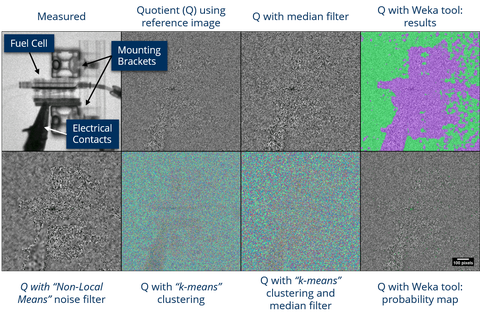
Figure 5-1: Reference image for starting measurement of water accumaulation of an air-breathing fuel cell
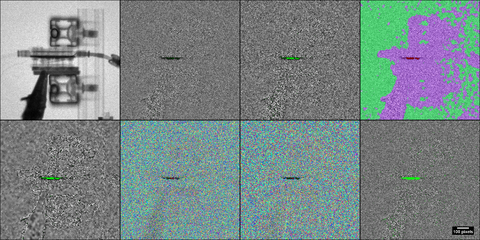
Figure 5-2: After a couple of minutes the first signs of water can be extracted
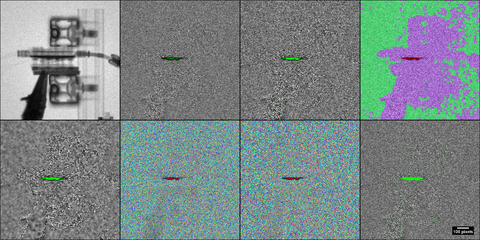
Figure 5-3: Further accumulation is destinguishable with respect to the former radiograph!
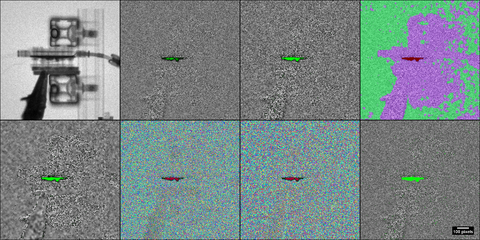
Figure 5-4: Finally, the fuel cell is getting close to be flooded
Technical details of the set-up
Camera (Andor iKon-M)
CCD-detector size: 1024x1024 px
Pixel size: 13.312 mm
Cooling: down to -70 °C
Preamplifier gain: up to a CCD sensitivity of 1.2 el./(A/D) at a noise of 2.8 el.
Set-up
FOV: 90x90 - 155x155 mm
Reproduction scale at FOV 136x136 mm: 1 px = 0.133 mm
Conducting Experiments
Since the contrast depends on the materials of the sample and the exposure time for each radiograph, no general estimation for the time needed can be made. Therefore, please don’t hesitate to contact us. We will gladly provide you with more information upon request.
References
[1] J. Schindelin et al., “Fiji: An open-source platform for biological-image analysis,” Nature Methods. 2012, doi: 10.1038/nmeth.2019.
[2] I. Arganda-Carreras et al., “fiji/Trainable_Segmentation: Trainable_Segmentation-3.2.24,” Mar. 2020, doi: 10.5281/ZENODO.3692971.
C. Lange, N. Bernt, "Neutron imaging at the low flux training and research reactor AKR-2", Nuclear Instruments and Methodes in Physics - Research Section A - Volume 941 Oct. 2019, doi:10.1016/j.nima.2019.06.033, URL https://www.sciencedirect.com/science/article/abs/pii/S0168900219308733?via%3Dihub