Flotation of non-metallic inclusions in liquid metals
Contact: Dr.-Ing. Tobias Lappan
Dr.-Ing. Sven Eckert
Funding: German Research Foundation (Deutsche Forschungsgemeinschaft, DFG)
Project no. 284002692: FLOTINC – Inclusion interactions during flotation in liquid metals
Motivation and objectives
Metallurgy aims to produce high-performance structural materials, such as aluminium alloys or steels, in a cost- and energy-efficient way. To this end, inclusion cleanliness is a major challenge, since it strongly influences the mechanical performance of metallic materials, can significantly reduce the weight and improve the quality of the final product. From the material-scientific point of view, non-metallic inclusions, typically having a mean diameter of around 10 µm, act as defects in the metallic microstructure, thus impairing the material properties. In steel production, ladle refining is commonly used to deplete the population of inclusions, particularly to reduce their concentration and control the size of the biggest remaining inclusions. Heated up above the melting temperature, the metal bath is stirred by gas bubbles, which are generated by chemical reactions in the ladle or are injected into the ladle additionally. Because of the bubble-induced turbulence, the probability of collisions between inclusions is increased and bigger inclusion aggregates are formed. Inclusions attached to bubbles are floated up towards the free surface of the metal bath, get adsorbed in the slag and can finally be removed.
Despite their critical impact on metallurgical processing, aggregation mechanisms and interactions of inclusions in liquid metals are still not fully understood nor adequately captured by correlations that could be used in process modelling and design due to their complexity. This lack of knowledge furthermore results from the complexity of experiments with liquid metals as well as from the multi-scale nature of the problem, which makes it impossible for state-of-the-art simulations to capture all physics at once.
The FLOTINC project has addressed these two bottlenecks by an innovative multi-scale concept dedicated to the fundamental aspects of the aggregation dynamics of inclusions during flotation. This includes developing original experiments with low-melting metals, namely gallium-based alloys, which are liquid at room temperature. The goal of these experiments was to investigate the behaviour of inclusions, represented by small model particles, under conditions that so far have not been reported in the literature. Radiographic imaging with X-ray and neutron radiation was used to provide in-situ visualisation of liquid metal multi-phase flows and for studying bubble–particle and particle–particle interactions. In this regard, a substantial challenge was to find appropriate material pairings that allow to perform radiographic measurements with an acceptable contrast-to-noise ratio in the X-ray and neutron images.
Liquid metal experiments
Within the framework of the FLOTINC project, our experimental research work focused on radiographic imaging of multiphase flows with small solid particles (Fig. 1) in liquid metals. Two very similar radiographic techniques, but using different types of radiation, were employed to visualise and investigate particle-laden liquid metal flows in laboratory-scale experiments with state-of-the art radiography setups. While X-ray radiographic imaging was performed in our in-house X-ray laboratory, neutron radiography was conducted at the imaging instruments NEUTRA and ICON, which are operated at the Swiss spallation neutron source SINQ, Paul Scherrer Institute. Although neutron radiography was not part of the original project proposal, during the course of the FLOTINC project, radiography with thermal or cold neutrons instead of conventional high-energy X-rays was identified and established as an additional imaging method for studying particle motions in liquid metal flows.
Beyond the focus on inclusions in liquid metals, radiographic imaging with both, X-rays and neutrons, was also applied to multiphase flow experiments with particles in liquid foam and froth. Their flow behaviour and applications are clearly different from that of liquid metals. However, the challenge of measuring motions of single particles within different optically opaque fluids is very similar. In this regard, to leverage synergies, the approach of image processing as well as the further data analysis were developed and improved in parallel for experiments with liquid metals as well as liquid foam and froth.
The radiographic measurements of particle motions in liquid metal flows are based on the principle of transmission imaging. This requires particles with significantly higher attenuation for X-rays or neutrons than the liquid gallium alloys, which served as model liquid metals in the laboratory-scale experiments at room temperature. To that effect, heavy metals such as lead (Pb) or alloys with high lead content (e.g. Pb90Sn10) as well as tungsten (W) or tungsten carbides (e.g. WC-Co) were identified as particle materials suitable for X-ray radiography. The rare-earth metal gadolinium (Gd), gadolinium oxide (Gd2O3) or gadolinium-rich composite particles are best possible candidates for neutron radiography. Such model particles were first classified by dry sieving into the required particle size ranges, then mixed with the liquid metal to form a suspension, and finally used for the X-ray or neutron radiographic studies.
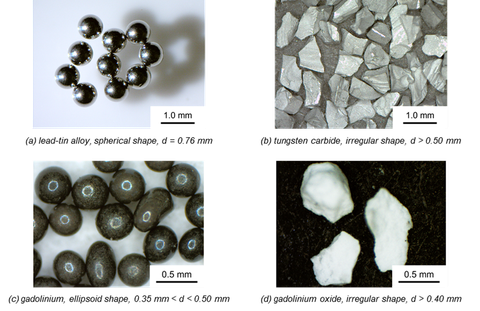
Fig. 1: Stereomicroscopic images of the model particles used for (a), (b) X-ray and (c), (d) neutron radiographic measurements of particle motions in liquid metal flows.
X-ray radiography
For the X-ray radiographic measurements in liquid metal flows, particles based on the heavy metals tungsten and lead were used in the eutectic gallium-indium-tin alloy (GaInSn). Theoretically, these particles are projected with the maximum X-ray image contrast if the X-ray beam energy matches with the element-specific adsorption edges. The radiographic experiments were performed using the continuous X-ray bremsstrahlung spectrum. Its energy corresponding to the maximum intensity is estimated, depending on the X-ray tube voltage, by means of Dauvillier’s law. Model particles of 0.5 mm < d < 1.0 mm in diameter have a sufficient contrast-to-noise ratio in the X-ray image sequences, which were acquired with the image pixel size dpx = 0.1 mm and the exposure time Te = 5 ms corresponding to the maximum imaging frame rate fimg = 200 fps. For X-ray radiography, the particle size has to be larger than the image pixel size in order to achieve an acceptable image contrast between the model particles and the surrounding liquid metal based on their X-ray attenuation coefficients.
The X-ray radiographic visualisation of the W- or Pb-based model particles in bubble-driven liquid metal flows is limited to a certain thickness dl along the X-ray beam direction. To achieve a sufficient image contrast for the particles, the thickness of the liquid metal has to be rather small. However, X-ray imaging of the bubbles requires a significant attenuation of the X-ray beam when penetrating the liquid metal. In order to visualise particles and bubbles in a liquid metal simultaneously by means of X-rays, the thickness dl is limited to less than 10 mm.
Generally, W- and Pb-based model particles are subjected to a strong tendency to settle in liquid metal flows. Compared to the liquid metal alloy, the particles’ higher X-ray attenuation coefficients are linked to higher mass densities, yielding significant terminal velocities of such particles in the liquid metal. Consequently, X-ray radiographic measurements visualised the counterflow of ascending gas bubbles and descending solid particles in the liquid metal. The motion paths of both, bubbles and particles, were measured and analysed simultaneously using tracking algorithms.
The X-ray radiographic experiments with particle-laden liquid metal flows driven by bubble injection provide visual insights into effects of bubble-particle interactions, such as collisions between bubbles and particles in the liquid metal. Depending on the wetting characteristics of the particle surfaces, these bubble-particle collisions possibly lead to attachments and detachments of particles at the bubble surfaces. In the radiographic flow experiments with the lead-tin spheres that are well-wetted by the liquid gallium-indium-tin alloy, collisions between argon bubbles and these spheres were not observed to result in stable bubble-particle attachments (Fig. 2).
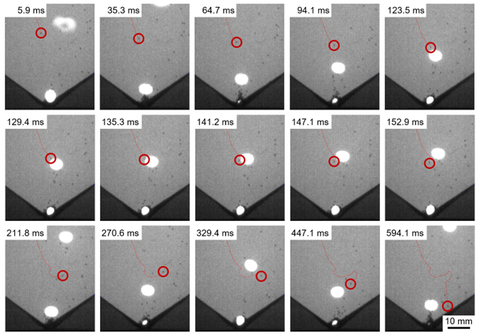
Fig. 2: X-ray image sequence of spherical lead-tin alloy particles (d = 0.76 mm) and argon bubbles in the liquid gallium-indium-tin alloy of 3 mm thickness along the X-ray beam direction. The X-ray images are superimposed with the previous motion path and the current position of one individual particle that approaches and collides with a bubble, but does not attach to the bubble surface as the particle surface is well wetted by the liquid metal.
Neutron radiography
For the neutron radiographic measurements in liquid metal flows, particles based on the rare-earth metal gadolinium (Gd) were used in a hypoeutectic gallium-tin alloy (GaSn). Theoretically, because of the outstanding high neutron attenuation coefficient, small gadolinium particles of only 0.05 mm in diameter reduce the neutron beam intensity by more than 99.9 %, thus appearing neutron-opaque with a maximum neutron image contrast. In the radiographic experiments, larger model particles of 0.3 mm to 0.5 mm in diameter have a sufficient contrast-to-noise ratio in neutron image sequences, which were acquired with the image pixel size dpx = 0.1 mm and the image exposure time Te = 10 ms corresponding to the maximum frame rate fimg = 100 fps. For neutron radiography, the particle size has to be larger than the image pixel size because of the intense image noise in the neutron radiographs acquired at this short image exposure time.
The neutron radiographic visualisation of the Gd-based model particles in liquid metal flows is significantly affected by the image noise. Also, bubbles in liquid metals are imaged with considerably lower contrast-to-noise ratio than by X-ray radiography. Using the neutron imaging instruments NEUTRA and ICON at the Paul Scherrer Institute, the inherent neutron flux is the crucial parameter limiting the spatial and temporal resolution of the neutron image sequences. Compared to the thermal neutrons at NEUTRA, the ICON instrument provides a higher cold neutron flux by means of a larger beam aperture, thus enhancing the contrast-to-noise ratio to a certain extent. It should be noted that NEUTRA and ICON are among the leading neutron radiography instruments regarding the neutron flux that is technically available; they are operated in a continuous mode and provide an almost homogenous illumination in an appropriate field of view for imaging flow measurements.
In contrast to the heavy W- and Pb-based model particles for X-ray radiography, the model particles from gadolinium or gadolinium oxide have a bulk density that is close to the mass density of the liquid metal alloy. Because of their low Stokes number, these particles are most suitable as neutron-opaque tracer particles for neutron radiographic measurements and flow analysis by applying particle image velocimetry (PIV) algorithms, which are well-established for optical measurements in transparent fluids.
The neutron radiographic experiment of the particle-laden liquid metal flow around a cylindrical obstacle in a 3 mm flat channel was motivated by a single rising bubble. As shown in Fig. 3, this liquid metal loop was driven by means of an electromagnetic induction pump, which allows the flow velocity and thus the Reynolds number to be adjusted over a wide range. Using the gadolinium oxides particles as tracers in the liquid metal flow, neutron radiography provides visual insights into the flow field up- and downstream the cylinder. Distinguishing and tracking of individual small particles in the cylinder’s wake flow, particularly prior to the formation of larger particle agglomerations, is challenging because of the intense neutron image noise. However, the fluid flow in the wake of a cylinder is characterized by a significantly lower magnitude of the local velocity. Assuming shear flow conditions in the wake region, individual particles with comparatively long residence times were identified to collide with each other.
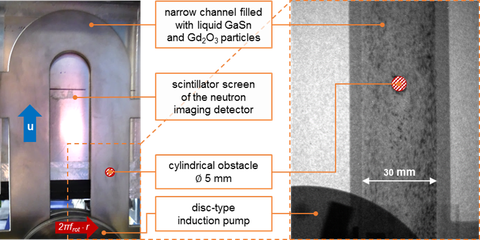
Fig. 3: Photograph of the experimental arrangement in front of the scintillator as part of the neutron image acquisition system, and a neutron radiograph of the liquid gallium-tin metal flow with dispersed gadolinium oxide particles in the straight channel section around the cylindrical obstacle, which is motivated by a single bubble.
Publications
- M. Birjukovs, P. Zvejnieks, T. Lappan, M. Sarma, S. Heitkam, P. Trtik, D. Mannes, S. Eckert, and A. Jakovics: Particle tracking velocimetry in liquid gallium flow around a cylindrical obstacle. Experiments in Fluids, 63(6):99, 2022, DOI: 10.1007/s00348-022-03445-2.
- T. Lappan: X-ray and neutron radiography of optically opaque fluid flows: experiments with particle-laden liquid metals and liquid foams, Dissertation, Technische Universität Dresden, 2021.
- T. Lappan, M. Sarma, S. Heitkam, D. Mannes, P. Trtik, N. Shevchenko, K. Eckert, and S. Eckert: X-ray and neutron radiographic experiments on particle-laden molten metal flows. In: J. Le, et al. (Eds.), Materials Processing Fundamentals 2021, pp. 13-29, Springer International Publishing, 2021, DOI: 10.1007/978-3-030-65253-1_2.
- T. Lappan, M. Sarma, S. Heitkam, P. Trtik, D. Mannes, K. Eckert, and S. Eckert: Neutron radiography of particle-laden liquid metal flow driven by an electromagnetic induction pump. Magnetohydrodynamics, 56(2/3):167–76, 2020, DOI: 10.22364/mhd.56.2-3.8.
- T. Lappan, A. Franz, H. Schwab, U. Kühn, S. Eckert, K. Eckert, and S. Heitkam: X-ray particle tracking velocimetry in liquid foam flow. Soft Matter, 16(8):2093-2103, 2020, DOI: 10.1039/C9SM02140J.
- S. Heitkam, T. Lappan, S. Eckert, P. Trtik, and K. Eckert: Tracking of particles in froth using neutron imaging, Chemie Ingenieur Technik, 91(7):1001–1007, 2019, DOI: 10.1002/cite.201800127.