Research
The cytoplasm is a mysterious jelly-like substance that sustains the biochemical reactions that are vital for life. Understanding its organization remains a major challenge.
Cells organize their cytoplasm by forming compartments. While membrane-bound compartments have been studied for decades, membraneless compartments, also called biomolecular condensates, are just beginning to be understood. Biomolecular condensates form through biophysical phase transitions and are crucial for cell organization and function. However, as cells age, their ability to form proper condensates declines, leading to aberrant condensates linked to aging and diseases like neurodegeneration and cancer. Consequently, current research is focused on understanding how condensates are connected to age-related diseases.
Our lab investigates the molecular mechanisms that control the organization of the cytoplasm using biochemical, biophysical, genetic, and cell biological methods. We study various model systems, including yeast and mammalian cells, to explore how biomolecular condensates respond to environmental stress. We propose that stress-induced condensates help cells to quickly adapt to changing conditions. Additionally, condensates create specialized microenvironments that enhance or inhibit key biochemical processes in cells, such as signaling, DNA damage repair, transcription, or translation.
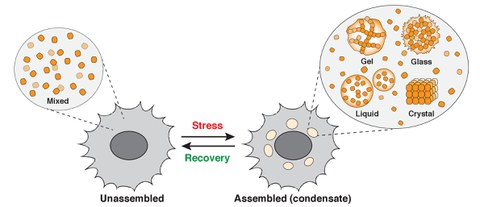
Alberti lab research focus - intracellular phase transitions
Research focus of the Alberti lab. The figure shows an idealized cell that transitions into a different physiological state upon stress. This transition is associated with changes in the organization of the cytoplasm and the formation of liquid- or solid-like condensates (gels, glasses, or crystals). In unstressed cells, the molecules are not interacting with one other and are well mixed (Unassembled). However, upon stress, they demix and assemble into biomolecular condensates with different material properties (Assembled). The process of condensate assembly has hallmarks of a phase transition.
The experimental work of the lab is currently focused on four areas of research:
1) The role of RNP granules in translation regulation
2) Regulation of DNA damage repair by condensate assembly
3) Biomolecular condensates as stress survival strategy
4) Age-related diseases and aberrant condensates
PROJECTS
Project 1: The role of RNP granules in translation regulation
Ribonucleoprotein (RNP) granules are biomolecular condensates composed of RNA binding proteins (RBPs) and RNA molecules. RNP granules exhibit dynamic features, such as fast exchange of components with their surroundings, high mobility of components within the granules, and an ability to undergo fission and fusion events and fast assembly or disassembly. Dysregulation of these features may have detrimental effects for cells and has been associated with neurodegenerative diseases. Recent findings also suggest that RNP granule dynamics are relevant for regulation of translation. One example is human stress-inducible RNP granules (stress granules), which form upon exposure to stressful conditions. Their assembly coincides with an arrest in global translation and stress granule disassembly is required to restore translation after stress. To date it is not clear if and how stress granules and other types of RNP granules regulate translation.
Stress granule assembly in human cells is preceded by polysome run-off, and the polysome-released mRNA then assembles into stress granules. The Ras GTPase activating protein-binding proteins 1 and 2 (G3BP1 and 2) are essential for stress granule assembly as they interact with free mRNA. We recently succeeded in rebuilding bona fide stress granules in the test tube using purified G3BP1 and RNA (Guillen-Boixet et al., 2020). We showed that the scaffold RNA-binding protein G3BP1 is initially in an auto-inhibited state, but when mRNA molecules are released from polysomes during stress, G3BP1 undergoes a conformational change that facilitates its assembly into networked RNA/protein condensates (Figure 1). Based on these findings we propose that condensation mediated by RBPs such as G3BP1 may be a general principle underlying RNP granule assembly.
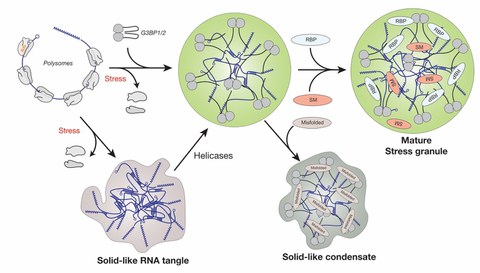
Project 1 - RNP granules in translation
Figure 1. Model for the assembly of stress granules in mammalian cells. Release of free mRNA from polysomes triggers the assembly of G3BP1 and 2 together with free mRNA into condensates. These condensates mature by recruiting additional factors such as RNA-binding proteins (RBPs) and signaling molecules (SM). RNAs can also aggregate via RNA-RNA interactions, which is modulated by RNA chaperones such as G3BP and ATP-driven helicases. Accumulation of misfolded proteins (misfolded) inside stress granules can trigger a transition to a less dynamic state that impedes stress granules dissolution.
In the future, we will continue to investigate the role of RNA condensates in translation regulation. Recent observations indicate that RNA-binding proteins such as G3BP1 are highly dynamic in reconstituted RNP granules, while mRNA is not. This suggests that mRNA dynamics inside condensates could be determined by trans RNA-RNA interactions that affect stress granule stability. Our immediate goals are to investigate 1) the role of RNA-RNA interactions in determining RNP granule stability and regulating mRNA availability and translatability, 2) the role of RNA chaperones such as G3BP in modulating RNA-RNA interactions and RNA assembly, and 3) the role of energy-driven helicases in modulating RNP granule assembly as well as mRNA availability and translatability.
Reference
J. Guillén-Boixet, A. Kopach, A. S. Holehouse, S. Wittmann, M. Jahnel, R. Schlüßler, K. K, I. R. E. A. Trussina, J. Wang, D. Matějů, I. Poser, S. Maharana, M. Ruer, D. Richter, X. Zhang, Y. T. Chang, J. Guck, A. Honigmann, J. Mahamid, A. Hyman, R. V. Pappu, S. Alberti und T. M. Franzmann (2020). RNA-Induced conformational switching and clustering of G3BP drive stress granule assembly by condensation. Cell, doi: 10.1016/j.cell.2020.03.049.
Project 2: Regulation of DNA damage repair by condensate assembly
DNA damage repair pathways protect the genetic information from environmental insults. In recent years, significant progress has been made in understanding DNA damage repair, revealing that cells have a variety of factors for this purpose. Cells can repair various DNA defects, with double-strand breaks (DSBs) being the most harmful. The two main DSB repair pathways are non-homologous end joining (NHEJ) and homologous recombination (HR). Choosing between NHEJ and HR involves complex signaling and molecular interactions, ensuring accurate DSB repair and maintaining genomic integrity.
Recent evidence supports the idea that DNA repair sites in cells are biomolecular condensates. Pioneering work by us showed that upon introducing a double strand break (DSB) into a DNA molecule, the DSB sensor protein PARP1 multimerizes and co-condenses with the broken DNA (Chappidi et al., 2024) (Figure 2). The co-condensates exert mechanical forces to keep DNA ends together and become enzymatically active for poly(ADP) ribose (PAR) synthesis. PARylation of PARP1 promotes the release of PARP1 from DNA ends and the recruitment of downstream effectors, such as the RNA-binding proteins FUS, which stabilizes broken DNA ends against separation, revealing a finely orchestrated order of events that primes broken DNA for repair. These findings offer a detailed molecular view of the organized assembly of repair factors at DSBs, revealing a new mechanism by which broken DNA ends are prepared for repair. We propose that condensates could hold the key to understanding the spatiotemporal regulation of DNA damage repair.
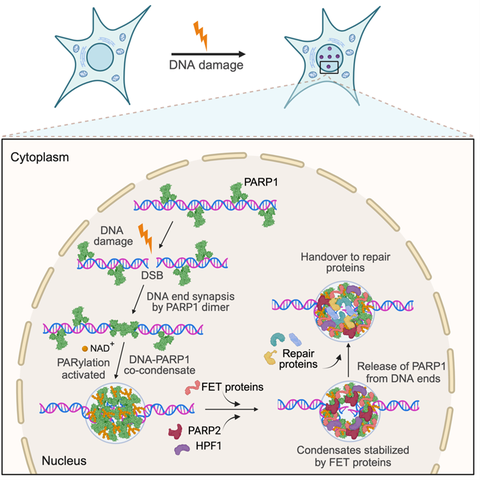
Project 2 - DNA damage pathways
Figure 2. A model for the assembly of DSB repair condensates. PARP1 co-condenses with DNA lesions to mediate the synapsis of broken DNA ends. Condensation around the lesion is driven by an ordered hierarchical assembly mechanism. The condensates exert balanced bidirectional condensation forces to prevent the separation of the DNA ends. PARP1-DNA co-condensation coincides with PARP1 activation and restricts poly(ADP) ribose PAR synthesis spatially to the damage site. PAR polymers recruit effector proteins, such as FET proteins, PARP2 and HPF1, and mediate the release of PARP1 from the DNA ends to prime condensates for repair. FET protein recruitment to primed condensates prevents condensate dissolution and promotes a transition from a rigid to a dynamic state, providing a functional role for the condensation properties of FET proteins.
In the future, we aim to study DNA repair through the lens of condensates to address key remaining questions related to spatiotemporal regulation of DSB repair. We aim to reveal molecular mechanisms governing break site detection and DNA end synapsis, reconstruct DNA repair condensates capable of repairing double-strand breaks, elucidate the role of condensates in pathway selection in DSB repair, and identify the impact of defective DNA repair condensates in the context of cancer. To achieve our goals, we will use biochemistry to reconstitute DNA repair systems from the bottom up using highly purified components, biophysics to quantitatively monitor condensate dynamics and properties, and cell biology to test the role of DSB repair condensates in physiology and disease.
References
N. Chappidi, T. Quail, S. Doll, L. T. Vogel, R. Aleksandrov, S. Felekyan, R. Kühnemuth, S. Stoynov, C. A. M. Seidel, J. Brugués, M. Jahnel, T. M. Franzmann, S. Alberti (2024). PARP1-DNA co-condensation drives DNA repair site assembly to prevent disjunction of broken DNA ends. Cell, 187(4):945-961.e18. doi: 10.1016/j.cell.2024.01.015.
A. Patel, H. K. Lee, L. Jawerth, S. Maharana, M. Jahnel, M. Y. Hein, S. Stoynov, J. Mahamid, S. Saha, T. Franzmann, A. Pozniakovski, I. Poser, N. Maghelli, L. Royer, M. Weigert, E. W. Myers, S. W. Grill, D. N. Drechsel, A. Hyman, S. Alberti. A liquid-to-solid phase transition of the ALS protein FUS accelerated by disease mutation. Cell, 162, 1066-1077, (2015) doi: 10.1016/j.cell.2015.07.047.
Project 3: Biomolecular condensates as stress survival strategy
How do cells adapt to stress? Stress induces massive changes in the organization of the cytosol. Upon exposure to stress, the budding yeast cytosol undergoes a phase transition from a liquid to a solid-like state (Munder et al., 2016). This phase transition is triggered by changes in physicochemical conditions such as fluctuations in cytosolic pH or temperature, which promotes assembly of specific proteins and RNAs into condensates. In this project, we test the hypothesis that cells use stress-induced condensates to detect changes in the environment and mount stress-specific responses. Recent key publications are:
- In 2018 we showed that the yeast polyU-binding protein (Pub1) assembles into condensates upon starvation or heat stress and that this is associated with cell cycle arrest (Kroschwald et al., 2018). Release from arrest coincided with condensate dissolution, which took minutes (starvation) or hours (heat shock). The different dissolution rates of starvation- and heat-induced condensates are due to their different material properties. Thus, different stresses induce condensates with distinct physical properties and thereby define different modes of stress adaptation and rates of recovery.
- In another study in 2018, we uncovered a novel function of the N-terminal prion domain of the canonical yeast prion protein and translation termination factor Sup35 (Franzmann et al., 2018). We showed that in stressed yeast, the Sup35 prion domain forms protective condensates via pH-regulated phase separation and subsequent gelation. Gelation promotes cell survival by rescuing the essential translation factor from stress-induced damage. This suggest that prion-like domains are protein-specific modifiers with chaperone-like functions that modulate protein phase behavior and protect proteins from damage.
- In 2020 (Iserman et al., 2020) we showed that condensate assembly by the yeast Ded1p helicase promotes a switch from making housekeeping proteins to making stress proteins on the level of translation. We showed that condensate assembly represses translation of housekeeping mRNAs with structurally complex 5’ UTRs, whereas stress mRNAs with structurally simple 5’ UTRs, including those encoding heat shock proteins, escape translational repression. These findings suggest that the assembly of heat shock granules by condensation is an adaptive response that downregulates the production of housekeeping proteins at the ribosome.
- In 2024 (Desroches Altamirano et al., 2024) we investigated the stress-specific function of the heterotrimeric translation initiation factor eIF4F, which is composed of the subunits eIF4G, eIF4E and eIF4A. We demonstrated that a heat-induced conformational rearrangement in the translation factor eIF4G dissociates eIF4A from eIF4F and promotes the assembly of translationally arrested mRNPs and heat shock condensates. eIF4A remains soluble during heat shock and promotes heat shock translation. This discovery allowed us to propose a comprehensive molecular model for the regulation of the heat shock response on the level of translation (Figure 3).
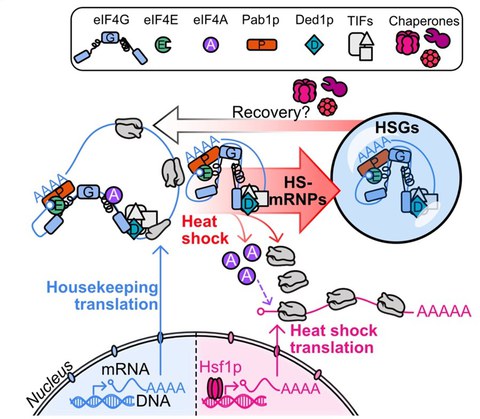
Project 3 - Condensates as stress survival strategy
Figure 3. Model for the regulation of the heat shock response on the level of translation. Heat shock induces the disassembly of the eIF4F complex, where eIF4G and eIF4E assemble into translationally arrested mRNA ribonucleoprotein particles (HS-mRNPs) and heat shock stress granules (HSGs), whereas eIF4A promotes heat shock translation. A conformational rearrangement of the thermo-sensing eIF4A-binding domain of eIF4G dissociates eIF4A and promotes the assembly with mRNA into mRNPs, which recruit additional translation factors, including Pab1p, Ded1p and eIF4E, to form multi-component condensates.
In the future, we will continue to characterize adaptive condensates that form in stressed cells. We hypothesize that stress-adaptive condensates regulate the structure and function of essential cellular proteins, thus allowing stressed cells to adapt to new environmental conditions, survive and recover from stress. Our immediate goals are to 1) dissect the functions of various stress-induced condensates, 2) investigate their mechanisms of assembly and disassembly, and 3) uncover the role of protein and RNA chaperones in regulating condensate assembly.
References
C. Desroches Altamirano, M. K. Kang, M.A. Jordan, T. Borianne, I. Dilmen, M. Gnädig, A. von Appen, A. Honigmann, T. M. Franzmann, S. Alberti (2024). IF4F is a thermo-sensing regulatory node in the translational heat shock response. Molecular Cell, doi: 10.1016/j.molcel.2024.02.038.
C. Iserman, C. D. Altamirano, C. Jegers, U. Friedrich, T. Zarin, A. Fritsch, M. Mittasch, A. M. De Jesus Domingues, L. Hersemann, M. Jahnel, D. Richter, U.-P. Guenther, M. W. Hentze, A. M. Moses, A. Hyman, G. Krämer, M. Kreysing, T. M. Franzmann und S. Alberti (2020). Condensation of Ded1p promotes a translational switch from housekeeping to stress protein production. Cell, doi: 10.1016/j.cell.2020.04.009.
S. Kroschwald, M. C. Munder, S. Maharana, T. M. Franzmann, D. Richter, M. Ruer, A. A. Hyman, S. Alberti (2018). Different material states of Pub1 condensates define distinct modes of stress adaptation and recovery. Cell Reports, 23, 3327-3339 doi: 10.1016/j.celrep.2018.05.041.
T. M. Franzmann, M. Jahnel, A. Pozniakovsky, J. Mahamid, A. S. Holehouse, E. Nüske, D. Richter, W. Baumeister, S. W. Grill, R. V. Pappu, A. A. Hyman, S. Alberti (2018). Phase separation of a yeast prion protein promotes cellular fitness. Science, 359, doi: 10.1126/science.aao5654.
M. C. Munder, D. Midtvedt, T. Franzmann, E. Nüske, O. Otto, M. Herbig, E. Ulbricht, P. Müller, A. Taubenberger, S. Maharana, L. Malinovska, D. Richter, J. Guck, V. Zaburdaev, S. Alberti (2016). A pH-driven transition of the cytoplasm from a fluid- to a solid-like state promotes entry into dormancy. eLife, e09347, (2016) doi: 10.7554/eLife.09347.
S. Kroschwald, S. Maharana, D. Matějů, L. Malinovska, E. Nüske, I. Poser, D. Richter und S. Alberti (2015). Promiscuous interactions and protein disaggregases determine the material state of stress-inducible RNP granules. eLife, doi: 10.7554/elife.06807.
Project 4: Age-related diseases and aberrant condensates
There are many debilitating age-related diseases that affect the nervous system, but the molecular causes of these diseases have remained largely unknown. In recent years, deficiencies in RNA metabolism mediated by RNA-binding proteins (RBPs) have come into the focus. RBPs are a highly abundant class of proteins that associate with RNAs to form ribonucleoprotein particles (RNPs). Recent studies suggest that defective RNP granules and alterations in RNA metabolism are linked to neurodegenerative diseases, including amyotrophic lateral sclerosis (ALS) and frontotemporal dementia (FTD). However, defective RNP granules only develop late in life, suggesting that young cells have mechanisms in place to control and prevent their formation. A major effort in the lab in recent years has been to investigate the role of aberrant condensates in causing age-related disease such as neurodegeneration.
Key publications in recent years have been:
- We demonstrated that the ALS-associated prion-like protein FUS forms dynamic condensates by phase separation (Patel et al., 2015). Importantly, condensates assembled from patient-derived FUS showed biophysical abnormalities and transitioned into an aberrant solid-like state. These findings suggested an explanation for why prion-like RNA-binding proteins are frequently associated with age-related diseases.
- In another study, we used extensive mutagenesis to identify a sequence-encoded molecular grammar underlying the driving forces for phase separation of FUS and related proteins (Wang et al., 2018). We found that phase separation of these proteins is driven primarily by interactions amongst tyrosine residues in prion-like domains and arginine residues in RNA binding domains. This work opened the door to predicting phase separation properties based on primary amino acid sequence.
- In 2017, we showed that misfolded proteins accumulate and aggregate within stress granules in human cells, decreasing stress granule dynamics, changing stress granule composition and triggering a transition to an aggregated state that can be rescued by chaperone recruitment (Mateju et al., 2017). This suggests that stress granule undergo a transition to an aggregated state driven by misfolded proteins that accumulate in aging cells.
- In 2018, we reported that the solubility of RNA-binding proteins such as FUS is regulated by RNA (Maharana et al., 2018). We showed that low RNA/protein ratios promote phase separation into condensates, whereas high ratios prevent condensate assembly. Reduction of nuclear RNA levels caused excessive phase separation and the formation of cytotoxic solid-like condensates in cells. Based on these findings, we proposed that the nucleus is a buffered system in which high RNA concentrations keep FUS soluble. Furthermore, changes in RNA levels or RNA-binding abilities of FUS could cause disease via aberrant phase transitions.
- In 2024, we reported a mechanism for the misfolding and aggregation of the ALS and FTD associated RNA-binding protein TDP-43. We demonstrated that TDP-43 aggregation requires a double event (Xiao et al., 2024): 1) up-concentration in stress granules beyond a threshold, and 2) oxidative stress (Figure 4). These two events collectively induce intra-condensate demixing, giving rise to a dynamic TDP-43 enriched phase within stress granules, which subsequently transitions into pathological aggregates. Based on these findings, we conclude that up-concentration inside condensates and exposure to environmental stress could be a general pathway for protein aggregation, with intra-condensate demixing constituting a key intermediate step.
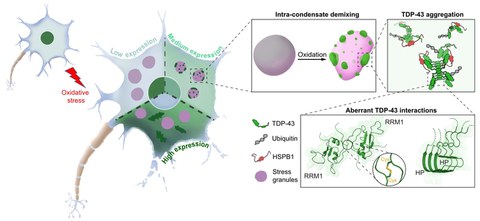
Project 4 - Aberrant condensates in disease
Figure 4: Model for the misfolding and aggregation of ALS-linked TDP-43 inside stress granules. TDP-43 aggregation requires a double event at intermediate physiological expression levels of the protein: 1) up-concentration in stress granules beyond a threshold, and 2) oxidative stress. Aggregation inside stress granules is preceded by an intra-condensate demixing event that is driven by the formation of disulfide bonds between cysteine residues that normally reside inside the folded RRM1 domain. Aggregation is further promoted by amyloid-like interactions that are mediated by the C-terminal helical patch (HP) region. Misfolded TDP-43 is modified with ubiquitin chains and associates with the HSPB1 chaperone.
In the future, we will investigate the link between biomolecular condensates and age-related disease. We are particularly interested in understanding 1) the role of condensates in promoting the emergence of disease, 2) the role of molecular chaperones and the protein quality control machinery in preventing the assembly of aberrant condensates, and 3) the role of age-related changes in metabolism and the emergence of aberrant condensates.
References
X. Yan, D. Kuster, P. Mohanty, J. Nijssen, K. Pombo-García, A. Rizuan, T. M. Franzmann, A. Sergeeva, P. M. Passos, L. George, S.-H. Wang, J. Shenoy, H. L. Danielson, A. Honigmann, Y. M. Ayala, N. L. Fawzi, J. Mittal, S. Alberti, A. A. Hyman (2024). Intra-condensate demixing of TDP-43 inside stress granules generates pathological aggregates. BioRxiv. doi: 10.1101/2024.01.23.576837.
J. Wang, J. Choi, A. S. Holehouse, X. Zhang, M. Jahnel, S. Maharana, R. Lemaitre, A. Pozniakovski, D. Drechsel, I. Poser, R. V. Pappu, S. Alberti, A. A. Hyman (2018). A molecular grammar underlying the driving forces for phase separation of prion-like RNA binding proteins. Cell, 174, 688-699 doi: 10.1016/j.cell.2018.06.006.
S. Maharana, J. Wang, D.K. Papadopoulos, D. Richter, A. Pozniakovsky, I. Poser, M. Bickle, S. Rizk, M. Jahnel, Y. T. Chang, P. Tomancak, A. A. Hyman, S. Alberti (2018). RNA buffers the phase separation behavior of prion-like RNA-binding proteins. Science, doi: 10.1126/science.aar7366.
D. Matějů, T. M. Franzmann, A. Patel, A. Kopach, E. E. Boczek, S. Maharana, H. O. Lee, S. Carra, A. Hyman und S. Alberti (2017). An aberrant phase transition of stress granules triggered by misfolded protein and prevented by chaperone function. EMBO Journal, 36 (12): 1669–1687. doi: 10.15252/embj.201695957
A. Patel, H. K. Lee, L. Jawerth, S. Maharana, M. Jahnel, M. Y. Hein, S. Stoynov, J. Mahamid, S. Saha, T. Franzmann, A. Pozniakovski, I. Poser, N. Maghelli, L. Royer, M. Weigert, E. W. Myers, S. W. Grill, D. N. Drechsel, A. Hyman, S. Alberti (2015). A liquid-to-solid phase transition of the ALS protein FUS accelerated by disease mutation. Cell, 162, 1066-1077, (2015) doi: 10.1016/j.cell.2015.07.047.